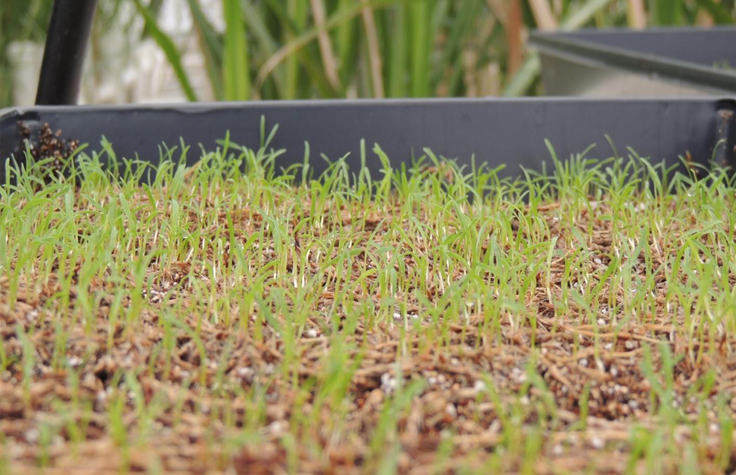
19 April 2023
Folk wisdom says that putting sugar in someone’s gas tank will ruin their car. Truth is, not only is that a myth, with a few extra steps, sugarcane can be turned into ethanol, a greener alternative to fossil fuels.
Sugarcane is a tropical grass in the same family as bamboo, wheat, maize, and rice. Three-quarters of all sucrose in our food comes from sugarcane (the rest comes from sugar beets); it’s grown in more than 80 countries, and by weight, more sugarcane is harvested than any other crop. It converts solar energy into chemical energy with nigh-unparalleled efficiency, and during its lifetime it removes so much carbon dioxide from the atmosphere that—if low-emissions practices are used in its harvesting and refinement—it can actually qualify as a carbon-neutral fuel source.
It didn’t start out so sweet. The two original wild species, Saccharum spontaneum and Saccharum officinarum, grown in India and New Guinea respectively, had limitations: the former had very thin stalks and the latter produced very little sucrose. Humans selectively bred the plant for thousands of years, to the point that the kind cultivated by the early 20th century was considered a whole new crop, resulting from the hybridization of those two species.
This “noble” cane’s thick stalks were saturated with sucrose, but it had lost so much genetic diversity it was vulnerable to pestilence from nearly every branch of the tree of life: The necrotic sugarcane yellow-leaf virus, the stunting bacteria Leifsonia xyli xyli, smut-causing fungal spores, and a moth whose larvae have such exclusive tastes we named it the “sugarcane borer.”
Farmers can control some of these nuisances with pesticides—which merely substitutes one problem with another. Cross-breeding the super-sweet noble cane with its hardier wild ancestor over years of research has produced some slightly more resilient cultivars, but to get the best of both worlds requires some next-generation science.
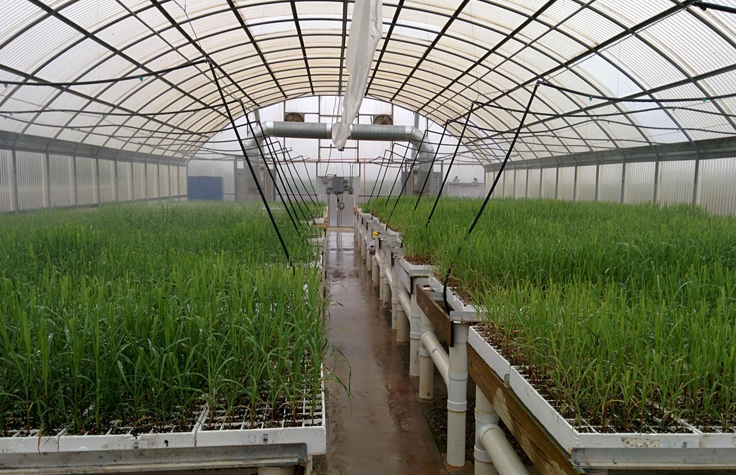
Cracking the decaploid genome
Enter the geneticists. For decades, the sugarcane breeding program at Texas A&M AgriLife Research and Extension Center in Weslaco has been creating varieties that are specifically adapted to the Rio Grande Valley’s 43,000 acres of sugarcane fields. Jorge Da Silva, a professor of the university’s Department of Soil & Crop Sciences, earned his PhD in plant genetic breeding at Cornell, and for 14 years before coming to the Lone Star State he served as a research scientist for Centro de Tecnologia Copersucar in Piracicaba, Brazil.
A&M couldn’t have asked for a better résumé. Bioethanol from sugarcane represents 17% of Brazil’s total energy supply; the country exports more sugar and—after the US—more ethanol than anyone else in the world; and CTC Copersucar is the country’s biggest producer of both. Da Silva has devoted his career to finding the best possible genes for this crucial crop.
“I love organic agriculture,” he says. “When I was a kid studying agronomy, I just wanted to be able to produce food without all the poisons. I realized that genetics would be the most powerful tool to reduce the amount of chemicals, just by breeding for plants that are resistant to pests, to microorganisms, to disease.” He also searches for genes that confer cold resistance, since Texas is already farther from the equator than any other sugarcane growing region. “Imagine if we could spread sugarcane to other parts of the US that have lower temperatures. That could be a big deal for the industry.”
Finding these genes hasn’t been easy, in part because the sugarcane genome is absurdly large. Humans have two copies of each chromosome; our genome is “diploid.” Plants often have more than two copies; their genome is “polypoid.” Da Silva explains: “A potato has four copies of each chromosome. Wheat has six. Sugarcane has ten.” Depending on the species and variety, the plant can have between 52 and 117 chromosomes total. If the human genome is a novel, the decaploid sugarcane genome is the unabridged Oxford English Dictionary. “It’s among the biggest genomes in the world. Sequencing all those chromosomes poses a big challenge for scientists.”
It also turns out that attempting to replicate the incredible growth and durability of the non-food wild cane comes with complications. “In this country, S. spontaneum is considered a noxious weed. It’s against the law to plant it because it’s very aggressive. It’s invasive; it becomes very hard to control. For me to do research with it, I had to get a permit from the USDA, and I still have a number of restrictions. Every year after I use the cane, I have to burn everything so it won’t propagate.”
But years of painstaking work is paying off. Da Silva’s research in Weslaco, comparing leaf chlorophyll content, leaf temperature, and relative water content in several different sugarcane genotypes subjected to a 90-day drought cycle, has generated a wealth of data about which genes are actually being expressed and controlling these traits. Genetic samples, or germplasm, from these crops have already been shared with the International Society of Sugarcane Technologies, and the US government recently awarded Da Silva’s program a grant to turn their attention to “energy cane”—sugarcane grown specifically to produce bioethanol.
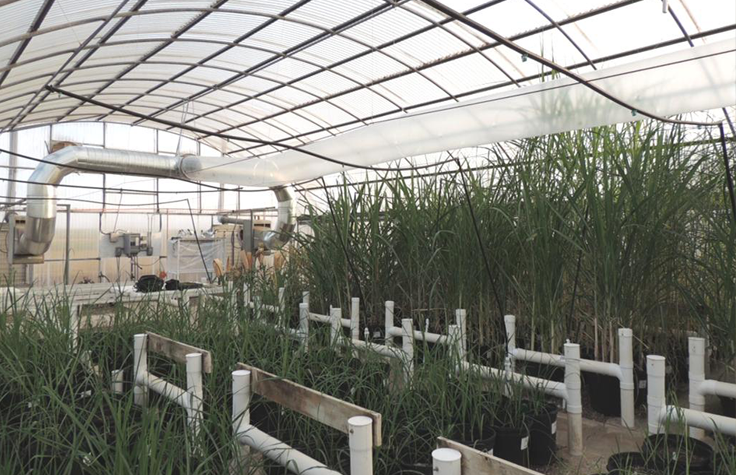
Food vs. fuel
When you can use the same crop for either food or fuel, how do you decide which to prioritize? Demand—and economic opportunity—for fossil fuel alternatives is high, but does making biofuels mean diverting food from someone’s plate, and potentially raising food prices?
In this case, if we process sugarcane the right way, we may be able to burn our cake and eat it, too.
To get edible sugar from the harvested plant, mill workers first crush the stalks in high-pressure rollers to squeeze out sucrose-rich juice. Then they boil the juice to remove water, and crystallize brown raw sugar in a centrifuge. That brown sugar can either be recrystallized into white sugar at a refinery, or fermented to produce bioethanol. This “first-generation” biofuel comes directly from an otherwise consumable food product.
But what about all those leftover dry, crushed stalks? Traditionally, they were simply burned to provide heat and, through steam power, electricity for the sugar mill. But something interesting happens if you heat this “lignocellulosic bagasse” in the absence of oxygen: Instead of burning, it undergoes thermal decomposition, or pyrolysis, that separates the lignin from the cellulose. The lignin can then be burned to help power the sugar mill, and the cellulose can be fermented into bioethanol without sacrificing any food.
This route comes with its own technological bottlenecks, and has yet to become economically viable, but it forms an important piece of the greener-fuel puzzle. That same lignocellulosic material can also be processed into composite forms of polyethylene and polypropylene—in fact, Illumina’s newest and most advanced sequencing systems, NovaSeq X and NovaSeq X Plus, use a biopolymer derived from sugarcane in their reagent kit cartridges, which can be recycled after use.
A plant with this many uses, and this many threats, ends up pulling its breeders in several directions at once. “We try to pyramid, as we call it, the resistance genes to biotic stresses like diseases and pests, but it’s hard to do that in addition to resistance to abiotic stresses like drought and cold,” Da Silva says. “We also want it to be high yield, and high sugar content.”
Ultimately, one factor affects both the feasibility and direction of breeding programs like Da Silva’s. “When I first came to Texas, I asked one of the sugarcane growers here, ‘What is the most important trait you want in a new variety?’ You know what he said? ‘Money in my pocket.’ Whatever we do that increases money in their pocket is welcome.”
With enough investment, next-generation sequencing could someday find the perfectly impervious, Swiss Army sugarcane that saves its growers money they would’ve otherwise spent on pesticides, that can be grown in a wider range, and—most importantly for future generations—is viable as both food and fuel.
Learn more about how Illumina is integrating sustainable solutions into our value chain, and supporting research into a more sustainable food supply through the Illumina Agricultural Greater Good Initiative.